Abstract
This review explores the pathophysiology of status epilepticus in adults, along with its incidence and key clinical considerations. We present a comprehensive treatment approach, emphasizing both emergent and urgent therapies. Additionally, we examine the role of newer agents such as clobazam and brivaracetam. This review also includes dosing strategies and potential drug-drug interactions of commonly used antiseizure medications in critically ill patients. Treatment strategies for refractory and super-refractory status epilepticus are also discussed. Finally, we provide a practical algorithm for a structured management approach, along with dosing and titration guidelines for sedatives following seizure cessation.
Keywords: Status epilepticus, pharmacotherapy, antiseizure medications
Introduction
Definition and Clinical Considerations
Status epilepticus (SE) is a medical emergency requiring immediate intervention to prevent long-term consequences arising from sustained seizure activity. Historically, SE was defined as seizure activity or the occurrence of two or more seizures without recovery of consciousness, lasting beyond five minutes. However, in 2015, the International League Against Epilepsy (ILAE) and the Commission on Epidemiology introduced a precise operational definition, incorporating two critical time points: T1 and T2 (1).
•T1 (Seizure Onset): For bilateral tonic-clonic (convulsive) SE, treatment should commence at 5 minutes, as seizures are unlikely to resolve spontaneously. In focal SE, with or without impaired consciousness, this threshold extends to 10 minutes.
•T2 (Risk of Neuronal Damage): This marks the maximum time window for effective seizure control to prevent long-term complications. For convulsive SE, the threshold is 30 minutes, while for focal SE, it is 60 minutes.
This definition underscores the necessity of prompt treatment to mitigate the risks associated with SE. Status epilepticus arises from either the failure of mechanisms responsible for seizure termination or the activation of mechanisms leading to abnormally prolonged seizures. After T1, seizure activity generally requires medical intervention to halt progression. Beyond T2, the risk of significant neuronal damage increases, necessitating aggressive management to prevent irreversible harm.
The ILAE’s operational framework for SE emphasizes the importance of timely recognition and intervention to reduce the risk of lasting neurological consequences (1).
Incidence
The global incidence of SE varies significantly across different regions and populations. According to a meta-analysis, the pooled crude annual incidence rate of SE is approximately 12.6 per 100,000 persons (2). The incidence of all types of SE in the USA ranges from 18.3 to 41 per 100,000 people per year. The incidence of convulsive SE (CSE), specifically, has increased from 3.5 per 100,000 in 1979 to 12.5 per 100,000 in 2010 (3). The goal of therapy is the rapid termination of both clinical and electrical seizure activity, since appropriate and timely therapy of status epilepticus reduces the associated mortality and morbidity. Delayed treatment can lead to neuronal injury, irreversible damage to vulnerable regions in the hippocampus, thalamus, and neocortex, and in some cases, brain tissue hypoxia, and increased intracranial pressure.
Risk Factors
Common causes for status epilepticus have been listed in Table 1 below. In children, the most common causes for status epilepticus are remote structural disease, acute symptomatic disease, and febrile seizures. In adults, the most common causes for status epilepticus are acute symptomatic disease such as stroke and metabolic derangements (4, 5).
Table 1. various causes leading to status epilepticus |
Acute symptomatic disease |
- Traumatic brain injury (TBI) - Acute ischemic stroke - Intraparenchymal hemorrhage - Metabolic derangements (hypoglycemia, hyponatremia) |
Remote structural disease |
- Encephalomalacia - Cerebral dysgenesis - Mesial temporal sclerosis |
Antiseizure drug withdrawal/non-compliance |
Febrile seizures |
Central nervous system (CNS) infections |
Autoimmune encephalitis |
Idiopathic/cryptogenic |
Pathophysiology
During SE, typical seizure termination mechanisms fail, or abnormal excitatory mechanisms are activated, resulting in prolonged seizure activity. As seizures persist, changes in neurotransmitter receptor dynamics progressively reduce the efficacy of termination strategies. A retrospective study in adults found that 80% of patients treated within the first 30 minutes of seizure activity experienced successful seizure termination, whereas this response rate dropped to less than 40% after 2 hours of seizure activity (6). Animal studies further demonstrate that prolonged seizures induce changes in neuronal membrane receptors, making seizure activity increasingly resistant to termination over time.
At the molecular level, benzo-diazepam sensitive synaptic gamma-aminobutyric acid, g-aminobutyric acid (GABA, gamma sub-unit), is internalized during prolonged seizures, leading to a reduction in membrane receptor density. This poses a challenge for treatment particularly with benzodiazepines, which are the first-line therapy for seizure termination. Conversely, prolonged seizure activity promotes the translocation of N-methyl-D-aspartate (NMDA) receptors to the synaptic membrane, increasing excitatory signaling. These receptor changes can occur within as little as 5 minutes of seizure onset. Figure 1 explains additional factors that exacerbate ongoing seizure activity, including the release of peptides, neuroinflammation, and the breakdown of the blood-brain barrier, all contributing to the increasing difficulty of terminating seizures over time (7).
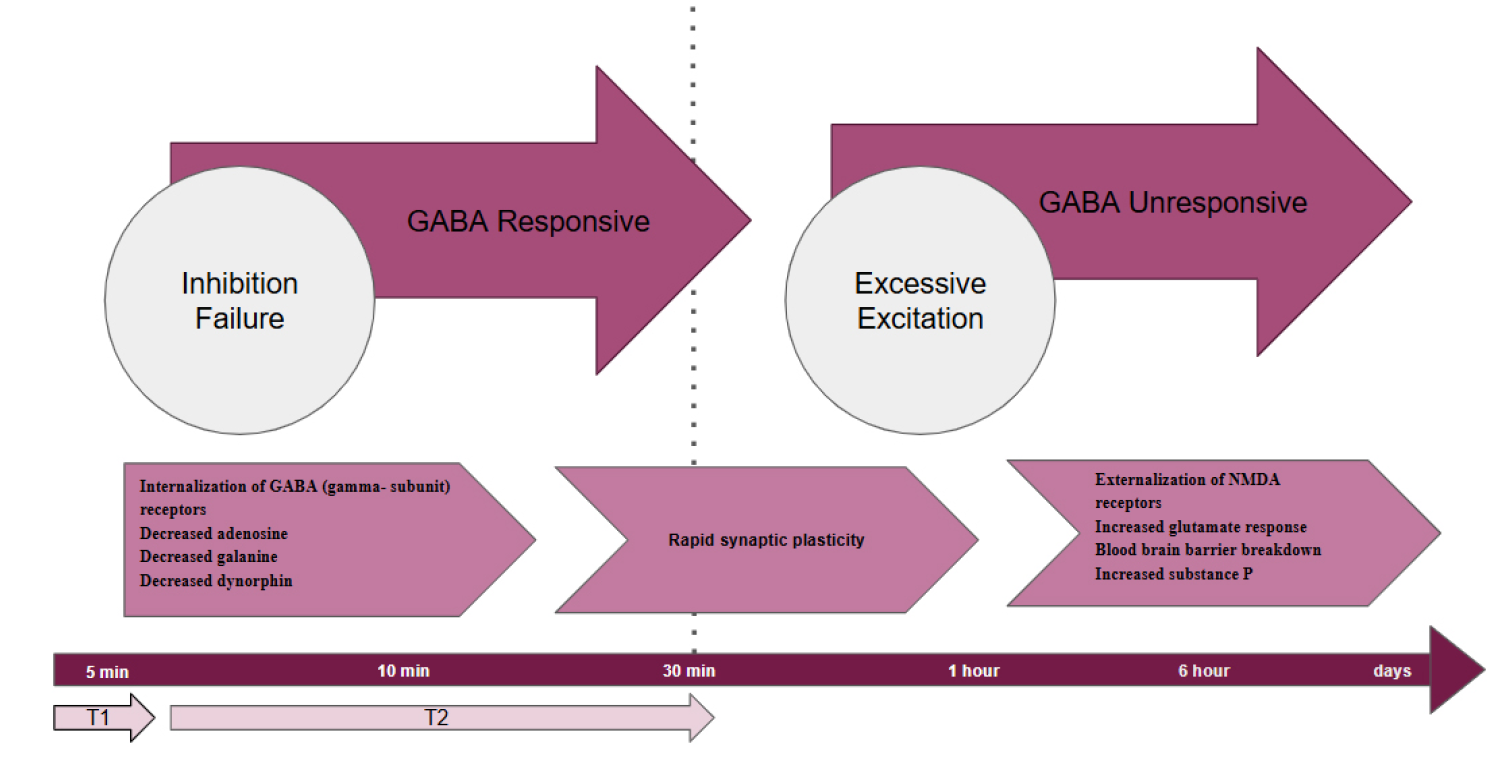
During convulsive SE, significant physiological changes occur, including alterations in heart rate, blood pressure, respiratory rate, blood glucose levels, body temperature, and electrolyte balance. The repetitive muscle contractions associated with convulsions impose extreme metabolic demands on the body. As convulsions persist, a shift to anaerobic metabolism occurs, resulting in increased lactic acid levels. Animal studies suggest that compensatory mechanisms begin to fail after 20 to 40 minutes of continuous seizure activity. Inadequate ventilation leads to hypoxia, which, coupled with pulmonary edema, contributes to respiratory acidosis. Simultaneously, metabolic acidosis develops due to lactic acid accumulation. Prolonged convulsions can also result in hyperthermia and rhabdomyolysis (7).
Prolonged SE can cause neuronal injury through mechanisms such as hypoxia, hyperthermia, acidosis, and hypoglycemia. This damage may lead to hippocampal sclerosis, characterized by the loss of neurons in the dentate nucleus and pyramidal layer of the hippocampus. Hippocampal sclerosis can serve as a focal point for seizures and epilepsy, perpetuating a vicious cycle of recurrent seizures and further neuronal injury (8).
Treatment
First-line (emergent) therapy: Effective initial therapy, also known as emergent therapy, according to the Neurocritical Care Society (NCS), depends on multiple factors, including drug choice, dosage, and timing. Benzodiazepines are considered a first-line treatment, with lorazepam being the preferred option in hospitals due to its proven efficacy in clinical trials (9). Clinical trials have shown that IV lorazepam is more effective than IV phenytoin and at least as effective as phenobarbital or diazepam plus phenytoin. In an out-of-hospital setting, IV lorazepam has a slightly better response than IV diazepam and is comparable to intramuscular (IM) midazolam (10). IM midazolam enters the systemic circulation quickly, providing a seizure cessation action like IV lorazepam. This is a viable alternative when IV access is delayed (Table 2).
Table 2. First line (emergent) therapy (14,16) | |||||
Drug |
Mechanism of action |
Route/dose |
Maximum dose per treatment |
Pharmacokinetic pearls |
Adverse reaction |
Lorazepam |
GABA-A receptor agonist |
0.1 mg/kg up to 4 mg IV push over 2 minutes, if still seizing after 5 min, repeat x 1 |
4 mg IV per dose |
Hepatic (conjugation metabolism) to inactive metabolite |
Contains propylene glycol (caution with continuous infusion) |
Midazolam |
GABA-A receptor agonist
|
0.2 mg/kg up to 10 mg IM, IN, buccal or IV |
10 IV/IM/IN mg per dose |
Extensive hepatic (CYP 3A4) metabolism to an active metabolite Drug-drug interaction with CYP 3A4 inducer and inhibitor |
Caution in renal impairment with continuous infusion only Active metabolite will accumulate in renal failure
|
Diazepam |
GABA-A receptor agonist |
0.15 mg/kg up to 10 mg IV push may repeat x 1 0.20 mg/kg up to 20 mg PR |
10 mg IV per dose 20 mg PR per dose |
Rapid onset; hepatic metabolism by CYP 3A4 and CYP2C19 to an active metabolite
|
PR dose needs to be rounded to nearest 2.5 mg IV formulation contains propylene glycol (caution with continuous infusion)
|
GABA: gamma-aminobutyric acid, IV: intravenous, IM: intramuscular, IN: intranasal, PR: rectal |
Timely and appropriate dosing is crucial for effective benzodiazepine therapy in status epilepticus. A lorazepam dose of 0.1 mg/kg (maximum 4 mg) is effective, while 10 mg of IM midazolam is appropriate for patients over 40 kg, when IV access is unavailable. Concerns about respiratory compromise and airway management with benzodiazepines are countered by evidence suggesting a lower intubation rate in treated patients compared to those left untreated.
Second line (urgent) therapy: Although various antiseizure medications are available for urgent therapy in status epilepticus, no clinical trials definitively support one agent over another in terms of efficacy. Studies reveal suboptimal response rates, with less than 50% seizure cessation for phenytoin or phenobarbital, and variable responses for valproate (70-88%) and phenytoin (25-84%) (11, 12). More recently the ESETT trial showed no difference in efficacy when levetiracetam, valproate, or phenytoin were utilized as second line agent for the management of status epilepticus (13). In this study, status epilepticus was stopped in approximately 50% of patients in each treatment group. It is, therefore, important to consider individual medication and patient-specific factors when choosing the second-line agent. Traditional agents such as phenobarbital and (fos)phenytoin have limitations, including prolonged infusion times and risks such as hypotension, respiratory depression, and arrhythmias, often necessitating airway protection and cautious infusion rates to mitigate adverse effects. Propylene glycol in these drugs can cause toxicity, including severe metabolic acidosis. Fosphenytoin allows faster administration but is still constrained by cardiovascular risks and in vivo conversion time. In contrast, newer agents such as levetiracetam and lacosamide can be infused more quickly, offering practical benefits such as reduced monitoring time and faster therapeutic concentrations. They also have fewer drug-drug interactions. Finally, valproate with a broad mechanism of action may be helpful in controlling seizures as a second-line agent. Valproate should be avoided in those with thrombocytopenia, severe liver disease, or pregnancy or of childbearing age. Additionally, the combination of valproate and carbapenem should be avoided, as it results in subtherapeutic valproate concentrations (Table 3). Therapeutic drug monitoring should be performed when available to help guide therapeutic decision making (Table 4).
Table 3. Second line (urgent) therapy | ||||||
Drug |
Dosing LD MD |
Clinically relevant PK interactions with other ASM |
Approximate half Life (hr) in non-critically ill patients |
Dose adjustment in renal impairment (Maintenance doses) HD CRRT |
Dose adjustment in hepatic impairment (Maintenance doses) |
Comment |
Levetiracetam |
LD: 60 mg/kg over 10-15 min (max 4500 mg). MD: 1500-4000 mg/day divided in 2 doses |
NA |
6 |
HD: 50% removed; 500 mg-1000 mg daily with 250-500 mg in the evening dose post HD CRRT: depending on the dialysate flow rate and critical illness dose adjustment may not be necessary |
Not indicated |
New evidence for IV push dosing in doses as high as 4500 mg Avoid in patients with severe psychiatric medical history |
Lacosamide |
LD: 10 mg/kg IV over 5-10 min (max 400 mg). MD: 200-600 mg/day in 2 divided doses |
NA |
13 |
Reduce dose in severe renal impairment (CrCl < 30 mL/min); max 300 mg/day. HD: Administer up to 75% of the indication-specific maximum dose; administer a supplemental dose (up to 50%) after each hemodialysis session CRRT: depending on the dialysate flow rate and critical illness dose adjustment may not be necessary |
Consider dose reduction |
Monitor PR interval prior to initiation and while on therapy if on other PR prolonging agents such as dexmedetomidine |
Fosphenytoin/phenytoin |
LD: 20 mg PE/kg IV (max 1500 mg) MD: 100 mg IV every 8 hours |
Induces CYP 1A2, 2B6, 2C, 3A3/4. Generally avoid use with most CYP3A4 substrates. Coadministration with valproate displaces phenytoin from protein binding sites Induces metabolism of valproate. |
15 |
Not indicated |
Consider dose reduction |
May cause rash, fever, hypotension, or arrhythmias (more common with IV phenytoin than IV fosphenytoin). IV phenytoin formulation contains 40% propylene glycol; may cause metabolic acidosis. Only compatible in saline (unlike fosphenytoin)Severe tissue injury may occur with extravasation of IV phenytoin, including rare purple glove syndrome. |
Clobazam |
LD: 1 mg/kg (most case reports utilized 60-70 mg) MD:20-40 mg in 2 divided doses |
Clearances of valproic acid and primidone are significantly reduced in the presence of clobazam. Phenobarbital, phenytoin, and carbamazepine are associated with increased clobazam concentrations. |
40 hours (parent drug) 80 hours (active metabolite: N-desmethylclobazam) |
NA IHD has shown not to affect clobazam concentration based on a case report.
|
Given extensive hepatic metabolism dose adjustment should be considered. Maximum daily dose in mild-moderate hepatic impairment is 40 mg/day. **not studies in severe hepatic impairment** |
Concentrations of the active metabolite N-desmethylclobazam are 3 to 5 times higher in patients who are known CYP2C19 poor metabolizers compared to CYP2C19 extensive metabolizers. Dose adjustment is needed in patients who are poor CYP2C19 metabolizers. |
Perampanel |
LD: 12-32 mg MD: 4-12 mg nightly |
Phenytoin, carbamazepine, oxcarbazepine can reduce perampanel’s concentration. |
109 |
Not indicated although use is not studied in CrCl <30 mls/min or HD. |
Dose reduction should be considered; in mild liver impairment maximum daily doe is 6 mg/day In moderate liver impairment maximum daily dose is 4 mg/day *Use is not recommended in severe (child-pugh class C). |
Perampanel is a selective non-competitive inhibitor of the ionotropic a-amino-3-hydroxy-5-methyl-4-isoxazolepropionic acid (AMPA) receptor Life-threatening psychiatric behavior such as suicidal thoughts are reported with this agent. |
Valproate |
LD: 40 mg/kg IV; max 3000 mg. If still seizing, give additional 20 mg/kg IV (max 2000 mg) MD: 2000-4500 mg divided in 2-4 doses |
Phenytoin and valproate may displace each other from protein binding sites Valproate markedly inhibits lamotrigine metabolism leading to ↑↑ lamotrigine levels and risk of side effects including rash. |
12 |
Not indicated |
Caution in hepatic impairment. |
Highly plasma protein bound (up to 90%). May cause hyperammonemia encephalopathy (treated with L-carnitine supplementation), hepatotoxicity, thrombocytopenia, and platelet dysfunction Concurrent use with carbapenems (meropenem,doripenem, imipenem, ertapenem) may result in markedly decreased valproic acid plasma concentrations. |
Phenobarbital |
LD: 15-20 mg/kg IV x once, may repeat 5 mg/kg to 10 mg/kg Seizure MD: 2 mg/kg/day in divided doses |
Phenobarbital reduces serum concentration of carbamazepine Phenobarbital may decrease the serum concentration of phenytoin Valproate increases the serum concentration of phenobarbital and phenobarbital reduces the serum concentration of valproate. |
80 hours |
Not indicated unless Crcl <10 msl/min In patients with Crcl <10 mls/min, administer 50% of total daily dose **in HD, a supplemental dose of 50% of usual dose should be given after HD** frequent therapeutic drug monitoring may be necessary. |
No specific dose adjustment-higher accumulation is expected. |
Some dosage forms may contain propylene glycol Common adverse effects include hypotension, bradycardia, CNS depression, and dose related respiratory depression. |
Topiramate |
LD: 200-400 mg MD: 100-400 mg/day in 2-4 divided doses (reports up to 1600 mg/day) |
Use with zonisamide and other carbonic anhydrase inhibitors may worsen metabolic acidosis Use with caution with valproic acid may worsen high ammonia levels CYP 3A4 inducers can reduce topiramate concentration significantly. |
21 |
Reduce dose by ~50% HD: supplemental dose may be necessary |
Consider dose reduction. |
May cause metabolic acidosis; caution with propofol, acetazolamide, zonisamide and metformin May cause renal stones.
|
Brivaracetam |
LD: 50-200mg MD: 100-300mg/day in two divided doses |
Patients who are poor CYP 2C19 metabolizers or those also on CYP 2C19 inhibitors may need dose reduction. |
9 |
Contraindicated in patients undergoing dialysis, but can be used in patients with AKI. |
Consider dose reduction min 50 mg/day and max 150 mg/day. |
high-affinity synaptic vesicle glycoprotein 2A ligand that is structurally related to levetiracetam. |
ASM: anti-seizure medication, LD: loading dose, MD: maintenance dose, HD: hemodialysis, CRRT: continuous renal replacement therapy |
Table 4. List of urgent ASM and its drug monitoring including adverse drug reactions | ||
ASM |
Therapeutic drug monitoring (mcg/mL) |
Adverse drug reaction (ADR) monitoring |
Phenytoin |
Total: 10-20 *Corrected for albumin* Free: 1-2 |
Nystagmus, lethargy, coma, thrombocytopenia, gingival hyperplasia, rash,Steven Johnson syndrom and Liver Function Tests. Narrow therapeutic index; close drug monitoring is required. |
Phenobarbital |
20-40 Adults: Trough levels up to 80 mcg/mL are used in SE |
Drowsiness, hepatoxicity, rash - Steven Johnson syndrom, thrombocytopenia |
Valproic acid |
50-100 Adults: trough levels up to 175 mcg/mL are used in SE |
Drowsiness, thrombocytopenia, hyperammonemia, polycystic ovarian syndrome, teratogenic, hepatotoxicity |
Lacosamide |
2.8-18 (Test of compliance) |
PR interval prolongation - risk of atrioventricular block, bradycardia, balance & coordination difficulties |
Levetiracetam |
12-46 (Test of compliance) |
Aggression, psychosis, asthenia |
ASM: anti-seizure medication, SE: status epilepticus |
In recent years, novel antiseizure medications such as clobazam and brivaracetam have gained popularity. The exact mechanism by which brivaracetam exerts its antiseizure effects remains unknown, though it is a high-affinity ligand of synaptic vesicle protein 2A (SV2A).
Compared to levetiracetam, brivaracetam binds to SV2A with 10- to 30-fold greater affinity. Its rapid onset of action and availability in an intravenous (IV) formulation make it an appealing option for the treatment of SE, refractory SE (RSE), and super-refractory SE (SRSE). While animal studies suggest potential benefits of this agent in SE, clinical evidence remains limited. Phase III trials in epilepsy have shown that adding brivaracetam to ongoing levetiracetam therapy does not provide additional therapeutic benefit. Notably, patients who had not been exposed to levetiracetam responded better to brivaracetam. Among those previously treated with levetiracetam, efficacy was greater in patients who discontinued it due to adverse effects rather than due to insufficient response. Future randomized trials are needed to clarify the role of brivaracetam when co-administered with levetiracetam in SE (14).
Clobazam, a 1,5-benzodiazepine, enhances GABA-A receptor activity with greater selectivity for subunits involved in anxiolytic and anticonvulsant effects than for those mediating sedation. Its ease of administration, rapid onset, and favorable safety profile make it a viable option for SE treatment in patients with enteral access (14). However, given the limited clinical evidence, the potential role of this agent as an early add-on oral therapy for SE should be further explored through prospective randomized trials. Table 3 summarizes dosing and clinical pearls regarding these novel antiseizure medications.
Finally, the pathophysiology of status epilepticus highlights the importance of rapid seizure cessation to prevent neurological and metabolic complications. Prolonged status epilepticus can reduce the effectiveness of traditional treatments and increase the risk of refractory status epilepticus. Animal models indicate that benzodiazepine receptors undergo endocytosis after about 30 minutes, resulting in benzodiazepine refractoriness, while increased NMDA-glutamate receptor expression sustains an excitatory brain state and thereby elevates metabolic demands. This highlights the interest in starting anti-NMDA agents such as perampanel (enteral) or ketamine (intravenous) earlier in the course of status epilepticus therapy (15). Figure 2 demonstrates a practical approach to choosing antiseizure medications in status epilepticus. Individual patient factors such as organ function and drug-drug interactions should be prioritized when choosing the antiseizure medication(s). Table 5 summarizes important landmark clinical trials in urgent and emergent management of status epilepticus.
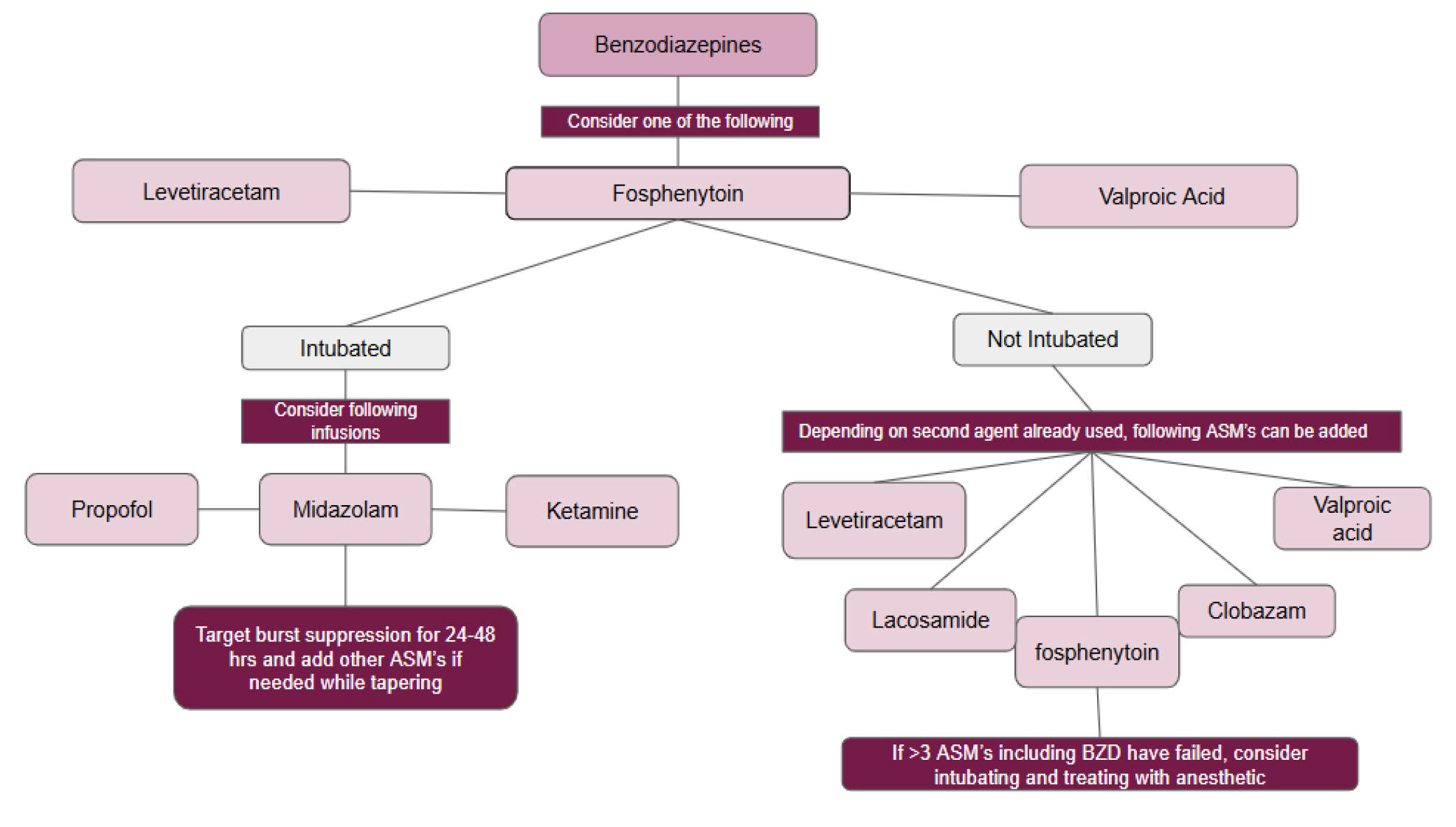
Table 5. Selected landmark trials in status epilepticus | ||||||
Trial |
n |
Intervention |
Primary outcome |
Secondary outcome |
Results |
Comments |
VA study (9) |
348 |
Diazepam (0.15 mg/kg) followed by phenytoin (18 mg/kg), lorazepam (0.1 mg/kg), phenobarbital (15 mg/kg), and phenytoin (18 mg/kg) |
Cessation of all motor seizure activity within 20 minutes of the start of the assigned medication. |
Adverse effects, Mortality and need for additional ASM* |
Lorazepam alone had the highest initial success rate of controlling status epilepticus (around 65%) Diazepam + phenytoin and phenobarbital alone followed in success rates (~55-58%).
Phenytoin alone had a lower success rate (~44%) |
Diazepam was given in combination with phenytoin, whereas lorazepam was given alone. Phenobarbital was also a stand-alone agent. This creates slight differences in how the regimens were compared. |
RAMPART (10) |
893 |
10 mg IM versed vs 4 mg IV lorazepam |
Cessation of Seizures before arrival to the ED* without the need for additional ASM |
Intubation, occurrence of recurrent seizures and complication |
Midazolam was non inferior to lorazepam in preventing prehospital status.
|
Study conducted in the US with trained paramedics, hence not generalizable for rural areas. |
ESETT (13) |
384 |
Second line agents in BZD* refractory status
Levetiracetam(60 mg/kg) vs Fosphenytoin (20 mg/kg) vs valproic acid (40 mg/kg)
|
Cessation of status epilepticus without the need for additional ASM within 60 min |
Safety, clinical improvement functional outcomes, mortality and morbidity |
No difference in primary and secondary outcomes between medications |
The study was under powered and the time frame of 60 minutes may not capture long term effectiveness of drugs and seizure recurrences |
VA: veterens affair, RAMPART: rapid anticonvulsant medication prior to arrival trial, ESETT: established status epilepticus trial, ASM: antiseizure medication, ED: emergency department, BZD: benzodiazepines |
Refractory Status Epilepticus and Super Refractory Status Epilepticus
RSE is defined as SE that persists despite at least two appropriately dosed parenteral ASMs, while SRSE is SE that persists either for at least 24 hours after the onset of continuous anesthetic medications (i.e., midazolam, propofol, pentobarbital, and ketamine) or during the weaning of these medications. However, it is important to note that, prolonged requirement for anesthetic coma was strongly associated with poor functional outcomes and functional decline. Mechanical ventilation was required in more than 90% of cases, one-third of which ultimately required tracheostomy. Longer duration of mechanical ventilation was associated with mortality. Additionally, cardiac arrhythmias requiring intervention, and pneumonia predicted poor functional outcome (17).
Recent case reports (18, 19) have shown that ketamine represents a safe and effective treatment option for refractory seizures without intubation, and thus has the potential to reduce morbidity associated with intubation in a carefully selected patient population. Early initiation may increase the likelihood of success. Table 6 summarizes appropriate dosing of anesthetics in this context.
Table 6. List of anesthetics that can be used in refractory and super refractory status | ||||
Drug |
Dosing: Loading dose Maintenance dose |
Side effects |
Tapering dose when seizure control or burst suppression is achieved for at least 24 hours |
Comments |
Propofol |
LD: 1-2 mg/kg MD: 30-200 mcg/kg/min |
Hypotension; Propofol-related infusion syndrome (PRIS) (80/kg/min > 48 hrs) respiratory depression |
20% reduction in every 3 hours |
Adjust calorie intake 1.1 kcal/mL. Monitor creatine kinase (CK), triglyceride, lactate, amylase, and lipase |
Midazolam |
LD: 0.2 mg/kg MD: 0.05-2.9 mg/kg/h |
Hypotension Respiratory depression |
50% decrease every 3 hours |
Tachyphylaxis with prolonged use Accumulation of active metabolite in renal impairment |
Ketamine |
LD: 0.5-1.5 mg/kg MD: 1-10 mg/kg/h |
Cardiac arrhythmias Emergence phenomenon Hyper/hypotension Metabolic acidosis |
20% reduction every 3 hours |
Cautious use in patients with history of severe cardiovascular disease Monitor LFTs
|
Pentobarbital |
LD: 5-15 mg/kg MD: 0.5-5 mg/kg/h
|
Cardiac depression Hypotension Metabolic acidosis Paralytic ileus Respiratory depression |
20% reduction every 3 hours |
Contains propylene glycol Monitor for hemodynamic adverse effects such as hypotension, bradycardia Potent CYP enzyme inducer |
Discontinuation of Anesthetics
Once under the anesthetic, patients are monitored on continuous EEG and targeted for burst suppression. This is aimed to last for at least 24-28 hours. Once achieved, the anesthetic can be weaned every 3 hours by 20-50% (Table 6) (20). If brief seizures progress to SE, anesthetic tapering should be stopped, and the dose should be increased to the prior, effective level. In addition, another anti-seizure medication can be added to aid in weaning anesthetic. Another 24-48 hours period of electrographic stability should be achieved before attempting an anesthetic withdrawal.
While deep sedation and burst suppression are well-described interventions for refractory status epilepticus, the specifics on weaning sedation are based on expert opinion, small observational studies, and standard critical care principles rather than large, dedicated randomized controlled trials. For now, clinicians rely on continuous EEG monitoring, gradual tapering, and strong maintenance antiseizure drug coverage to prevent rebound seizures and optimize outcomes.
Conclusion
SE presents a significant clinical challenge due to its potential for rapid progression to irreversible neuronal damage. The ILAE’s operational framework underscores the importance of early recognition and time-sensitive treatment. Delayed intervention increases the risk of mortality and long-term morbidity, evidenced by the steep decline in treatment efficacy beyond 30 minutes of seizure activity.
Recent insights into the pathophysiological mechanisms of SE provide a basis for targeted interventions. The role of receptor dynamics (e.g., GABA-A receptor internalization and NMDA receptor externalization) has informed the use of benzodiazepines and highlighted the need for alternative therapies in benzodiazepine-resistant cases.
Advances in ASM pharmacokinetics, newer agents like brivaracetam, clobazam, and perampanel, and adjunctive therapies like ketamine offer hope for better outcomes in refractory cases. However, the management of SRSE remains complex, requiring careful balancing of sedation, ventilation, and systemic support to minimize complications. Further research is needed to refine treatment algorithms for RSE and SRSE, identify biomarkers predicting treatment response, and develop neuroprotective agents to mitigate long-term neuronal damage.
In conclusion, managing SE requires a multidisciplinary approach, combining prompt intervention with an understanding of its complex pathophysiology. Standardized protocols and emerging therapies promise to improve patient outcomes, especially in refractory and super-refractory cases.
Footnotes
Authorship Contributions
References
- Trinka E, Cock H, Hesdorffer D, Rossetti AO, Scheffer IE, Shinnar S, et al. A definition and classification of status epilepticus--Report of the ILAE Task Force on Classification of Status Epilepticus. Epilepsia. 2015;56:1515-23.
- Lv RJ, Wang Q, Cui T, Zhu F, Shao XQ. Status epilepticus-related etiology, incidence and mortality: a meta-analysis. Epilepsy Res. 2017;136:12-7.
- Lu M, Faure M, Bergamasco A, Spalding W, Benitez A, Moride Y, Fournier M. Epidemiology of status epilepticus in the United States: a systematic review. Epilepsy Behav. 2020;112:107459.
- Trinka E, Höfler J, Zerbs A. Causes of status epilepticus. Epilepsia. 2012;53:127-38.
- Singh RK, Stephens S, Berl MM, Chang T, Brown K, Vezina LG, et al. Prospective study of new-onset seizures presenting as status epilepticus in childhood. Neurology. 2010;74:636-42.
- Mayer SA, Claassen J, Lokin J, Mendelsohn F, Dennis LJ, Fitzsimmons BF. Refractory status epilepticus: frequency, risk factors, and impact on outcome. Arch Neurol. 2002;59:205-10.
- Walker MC. Pathophysiology of status epilepticus. Neurosci Lett. 2018;667:84-91.
- Meldrum BS, Horton RW. Physiology of status epilepticus in primates. Arch Neurol. 1973;28:1-9.
- Treiman DM, Meyers PD, Walton NY, Collins JF, Colling C, Rowan AJ, et al. A comparison of four treatments for generalized convulsive status epilepticus. N Engl J Med. 1998;339:792-8.
- Silbergleit R, Lowenstein D, Durkalski V, Conwit R, Neurological Emergency Treatment Trials (NETT) Investigators. RAMPART (Rapid Anticonvulsant Medication Prior to Arrival Trial): a double-blind randomized clinical trial of the efficacy of intramuscular midazolam versus intravenous lorazepam in the prehospital treatment of status epilepticus by paramedics. Epilepsia. 2011;52:45-7.
- Misra UK, Kalita J, Patel R. Sodium valproate vs phenytoin in status epilepticus: a pilot study. Neurology. 2006;67:340-2.
- Agarwal P, Kumar N, Chandra R, Gupta G, Antony AR, Garg N. Randomized study of intravenous valproate and phenytoin in status epilepticus. Seizure. 2007;16:527-32.
- Kapur J, Elm J, Chamberlain JM, Barsan W, Cloyd J, Lowenstein D, et al. Randomized trial of three anticonvulsant medications for status epilepticus. N Engl J Med. 2019;381:2103-13.
- Farrokh S, Bon J, Erdman M, Tesoro E. Use of newer anticonvulsants for the treatment of status epilepticus. Pharmacotherapy. 2019;39:297-316.
- Claassen J, Hirsch LJ, Emerson RG, Mayer SA. Treatment of refractory status epilepticus with pentobarbital, propofol, or midazolam: a systematic review. Epilepsia. 2002;43:146-53.
- Farrokh S, Tahsili-Fahadan P, Ritzl EK, Lewin JJ, Mirski MA. Antiepileptic drugs in critically ill patients. Critical Care. 2018;22:153.
- Hocker SE, Britton JW, Mandrekar JN, Wijdicks EF, Rabinstein AA. Predictors of outcome in refractory status epilepticus. JAMA Neurol. 2013;70:72-7.
- Syed MJ, Zutshi D, Muzammil SM, Mohamed W. Ketamine to prevent endotracheal intubation in adults with refractory non-convulsive status epilepticus: a case series. Neurocrit Care. 2024;40:976-83.
- Kimmons LA, Alzayadneh M, Metter EJ, Alsherbini K. Safety and efficacy of ketamine without intubation in the management of refractory seizures: a case series. Neurocrit Care. 2024;40:689-97.
- Legriel S, Oddo M, Brophy GM. What’s new in refractory status epilepticus? Intensive Care Med. 2017;43:543-6.
Copyright and license
Copyright © 2025 The Author(s). This is an open access article distributed under the Creative Commons Attribution License (CC BY), which permits unrestricted use, distribution, and reproduction in any medium or format, provided the original work is properly cited.